Evidence of a synthetic biology revolution is mounting. Scores of startups have been introducing sustainable, bio-engineered fragrances, skin creams, polyurethane foam for alpine skis, biodegradable fast-food containers, and effective alternatives to nitrogen fertilizers. Corporate giants such as Unilever, Zara, and Adidas are unveiling collaborations with biotech firms to create petrochemical-free plastics, laundry soaps, sport-shoe materials, and strong, stretchable fabrics that, in the future, may even repair themselves.
Two major constraints, however, are preventing synthetic biology—syn-bio, for short—from going mainstream: cost and the limited availability of biomanufacturing capacity. Right now, most startups are working with contract biomanufacturing organizations that generate small quantities of new substances, making their output very expensive. What we really need are industrial-scale fermentation sites that cost-efficiently produce many kilotons of products. Those may require capital expenditures of $300 million to $400 million—far less than an oil refinery, but still a heavy investment.
It’s a bit of a Catch-22. Until there’s more capacity, it’s hard to bring down unit costs. And until the economics are there, few companies and investors want to build huge fermentation facilities. As a result, most venture capital still flows into biotech companies that are designing molecules used to make niche products for customers willing to pay premium prices.
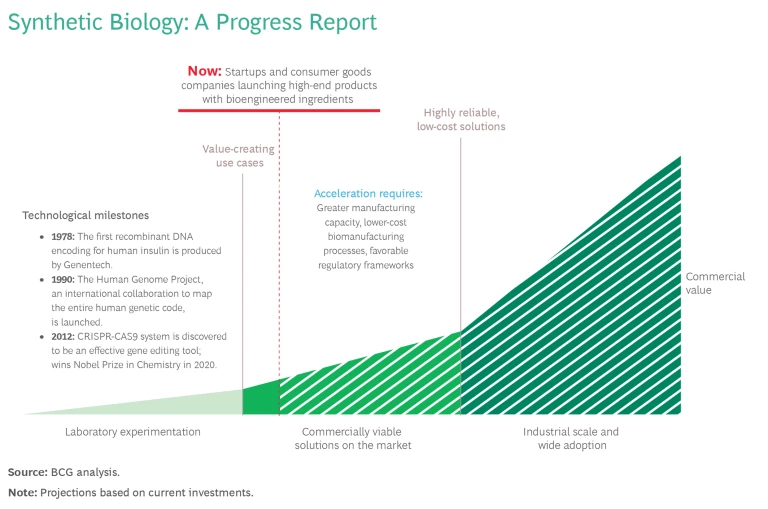
Syn-bio’s inflection point is nearing, however. The industry is being pushed by rapid technological advances that are enabling companies to create molecules of interest much faster, and in far greater variety. And it’s being pulled by growing demand for sustainable products across a range of industries. As a result, we’re starting to see a new generation of industrial-scale biomanufacturing facilities.
Syn-Bio’s Next Big Wave Is Hitting Now
Syn-bio is based on our understanding of the underlying design principles of every type of organism in nature, from microbes and plants to the human body. It originated in the pharmaceutical industry in the 1970s and 80s with the production of recombinant insulin enabled by genetic engineering, which continues to help us create therapies to cure people of diseases in ways that chemicals cannot. Decades later, syn-bio was deployed to make biofuels, although they have yet to reach true cost-parity with fossil fuels.
The latest—and most successful—wave of syn-bio is hitting now. New tools such as CRISPR-Cas9 allow us not only to change existing biological material but also to manipulate and redesign almost any organism. Within weeks, you can create and test millions of permutations of an organism to identify genetic aberrations that can lead to molecules of interest. And testing equipment is improving. These advances are dramatically shrinking development times and the cost of bringing syn-bio out of labs and closer to industrialization. Through a process called precision fermentation, it’s becoming theoretically possible to replicate any petrochemical-based product we use daily, including plastics, paint, and emollients for cleaning and skin care products.
Syn-bio essentially is about designing for sustainability while also potentially delivering unprecedented performance. The goal is to make better products that are also less harmful to the environment (that is, biodegradable) rather than dumped in landfills. Bioengineered foods and materials, sourced from renewable feedstock such as sugar, can have carbon footprints that are 30% to 70%, smaller than comparable products made with petrochemistry. If we can convert to different feedstocks—such as captured CO2 rather than sugar cane or corn—those footprints could shrink by 80% to 90%.
Syn-bio is being pushed by rapid technological advances, and it's being pulled by growing demand for sustainable products.
Harnessing Nature’s Building Processes
There are two basic types of application. One is “bio replacements,” or “bioidenticals”: copies of existing molecules, down to their atomic structure. The biggest market now is for bio replacements because they can go directly into existing industrial supply chains. Perfect Day has already brought sustainable dairy products to market in partnership with major food companies such as Nestlé and Mars. The big challenge, though, is to achieve cost parity with conventional products—while also tasting good and being better for the environment.
The other basic category is “bionatives,” based on entirely new molecules and materials that humans haven’t yet produced through chemistry. Pivot Bio, for example, has engineered microbes to transform nitrogen in the air into ammonia, a key plant nutrient. Its product is enabling thousands of farmers to use less nitrogen fertilizer, whose production generates several gigatons of CO2 emissions a year and pollutes water when it runs off fields.
A host of companies are developing alternative leather, packaging, fabrics, and plastics made from biological materials derived from algae, spider silk, and mycelium—dense, root-like networks of fungal fibers. In addition to being better for the environment, some of these nature-based materials have other valuable properties. Spider silk protein, for example, can be tougher than steel—yet remarkably flexible. Nike and Adidas are looking to use it in sports shoes.
Companies are also making progress in using bioengineered organisms and precision fermentation to replace base petrochemicals. Checkerspot, for instance, makes polyurethane resin derived from algae that is used to make the foam cores of skis.
Large Bioreactors for Base Chemicals Are on the Way
The next step is to produce kilotons or megatons of sustainable chemicals. That could be a decade away. But the first industrial-scale facilities are appearing. Solugen’s sizable wind-powered Bioforge in Houston, for instance, uses engineered enzymes to convert biomass from corn syrup into commercial chemicals. Among the five production sites of Chicago-based LanzaTech is a joint venture in Belgium with ArcelorMittal that has the capacity to produce 80 million liters of ethanol a year by using bacteria to ferment CO2 captured from factories.
Another encouraging sign is that competitors within the same industry are beginning to pool their financial firepower to commercialize bio-designed molecules that can become standard materials in their industry. Unilever, L’Oréal, and Kao, for example, are collaborating with San Diego-based Genomatica to scale up production of sustainable alternatives to palm oil, much of which currently is grown on cleared rainforests, for cleaning and personal care products. These kinds of collaborations can spread the risk of development while guaranteeing a market for the output.
Another encouraging sign is that competitors within the same industry are beginning to pool their financial firepower to commercialize bio-designed molecules that can become standard materials in their industry.
Governments are also increasing support. For example, the Bold Goals for US Biotechnology and Biomanufacturing, unveiled in early 2023, call for building domestic capacity. Among the goals are the replacement of 30% of all petrochemicals with biochemicals within two decades.
Once big biomanufacturing fermentation plants demonstrate their utility and become standardized, they can become an attractive asset class for institutional and private equity investors, who can then fund similar factories. As biomanufacturing’s financial risks decline, demand is likely to rise, paving the way for wide adoption. This could resolve the Catch-22—and mark the inflection point when syn-bio starts transforming entire industries.